Evaluating the Formation of Non-Enzymatic Oxidation Products of EPA and AA in a Population at Risk for Omega-3 Long-Chain Polyunsaturated Fatty Acid Deficiency
This article at a glance
A diet rich in omega-3 long-chain polyunsaturated fatty acids (LCPUFA), such as EPA and DHA, can reduce the risk for development of cardiometabolic disease, and attenuate the intensity of a range of chronic inflammatory conditions. The angio-protective, anti-inflammatory and anti-thrombotic properties of PUFA that contribute to risk reduction are mediated in part through their enzymatic conversion into an array of oxygenated lipid mediators that tone down inflammatory responses and help regulate several fundamental physiological functions such as blood pressure, lipid homeostasis, and hemostasis. Interestingly, some oxidation products of PUFA that are formed without the involvement of oxygenating enzymes have also been recognized to have biological activity, and can be detected in vivo. The phytoprostanes, isoprostanes (IsoPs) and neuroprostanes belong to such non-enzymatic oxidation products of alpha-linolenic acid, arachidonic acid (AA), eicosapentaenoic acid (EPA) and docosahexaenoic acid (DHA). These substances can be used to monitor the non-specific chemical oxidation of PUFA contained in membranes, and AA-derived isoprostanes (so-called F2-isoPs) are used as biomarkers of oxidative tissue injury. Augmented F2-isoP levels have also been correlated with risk factors for obesity and cardiometabolic disease, such as blood pressure and C-reactive protein (CRP) levels. Interestingly, isoprostanes derived from EPA (F3-IsoPs) and neuroprostanes derived from DHA do not seem to share the adverse effects of arachidonic acid-derived F2-isoPs, and may mediate protective effects in cells and tissues.
In the Inuit, a population of the Arctic, the absolute requirements for dietary EPA and DHA are higher than in most other human populations, since over the course of their evolutionary history their dietary pattern has been dominated by a high proportion of marine foods containing preformed EPA and DHA. The absence of a need to convert plant-derived α-linolenic acid to longer chain PUFA has rendered Inuit people highly dependent on preformed EPA and DHA in their diet for maintaining high tissue levels of omega-3 LCPUFA. From a perspective of understanding the effects of dietary insufficiency of EPA/DHA on health, the Inuit constitute an interesting human population to study the relationships between diet type, changes in fatty acid status, and the development of risk factors for disease in humans. In addition, a relatively recent shift away from traditional food and lifestyle over the last 50-100 years has led to an increased incidence of cardiometabolic disease in Canadian Inuit from a historical baseline level that was typically much lower than in most Western populations. The relationship and effect of diet and lifestyle, and the role of PUFA intake in particular, may be studied with higher clarity in the Inuit than in other populations. A cross-sectional study was recently carried out in 36 Canadian Arctic Inuit communities to gain insight into the relation between omega-3 status, EPA-derived F3-isoprostane formation and cardiometabolic risk factors, including F2-isoPs. The study was performed by Alkazemi and colleagues from the School of Dietetics and Human Nutrition and Centre for Indigenous Peoples’ Nutrition and Environment at McGill University, Québec, Canada, together with colleagues at Kuwait University, Kuwait, Vanderbilt University in Nashville, TN, and the University of Ottawa, Canada. A total of 233 Inuit adults (56% women) were studied with respect to dietary habits, physical activity, psychosocial factors, medical history, blood pressure, anthropometric indices, fasting blood lipids and various clinical indices. People with high CRP values (≥10mg/l), constituting 21% of the randomly selected individuals, had been excluded. The mean body mass index was 27.8 kg/m2, 34% were overweight, 30% were obese, and 29% had hypertension. Taken together, the study population can be considered at significant risk for developing, or is already displaying, cardiometabolic disease. Plasma F2-IsoP levels were on average at the high end of the concentration range considered normal, and 28% of participants had elevated levels of F2-IsoPs.
The researchers first assessed how various measured variables correlated to each other. F3-IsoPs were positively related to F2-IsoPs, suggesting that as the chemical oxidation of AA increases, so does that of EPA. However, the relation between isoprostane levels and selenium levels was completely different: at higher Se concentration, lower levels of F2-IsoPs were found, but F3-IsoPs levels were higher. The results suggest that the function of selenium, typically thought to support an antioxidant role through Se-dependent glutathione peroxidase, is differentially related to different isoprostanes. Older individuals were found to have higher F3-IsoP levels, but this did not correlate with any metabolic measures (blood lipids, CRP, blood pressure or fasting glucose). The ratio of F2-IsoPs to F3-IsoPs was strongly negatively related to F3-IsoP levels, which the authors suggest could mean that F3-IsoPs may affect F2-IsoPs formation. Although correlations do not signify any causal mechanisms, these results suggest that F3-IsoP formation shows some fundamental differences with F2-IsoP formation, and that a mere passive chemical oxidation of AA and EPA to the respective isoprostanes may be a too simplistic view. However, as expected, F3-isoP levels were positively associated with total omega-3 LCPUFA levels, and negatively associated with the ratio of AA to EPA levels in red cell membranes. To better quantify the association of omega-3 LCPUFA status with various other variables, such as isoprostanes, different fatty acids, Se, and the environmental contaminants Hg (total blood) and PCBs, study participants were stratified by low (<4.36%), medium (4.36-6.89%) and high (≥6.9%) levels of omega-3 LCPUFA in red blood cell membranes. Although no significant differences between F2-IsoP levels were found between tertiles, all other measured variables showed significant differences between at least one and the other two tertiles. Hg and PCB levels increased with increasing omega-3 LCPUFA levels. Se was higher in the highest tertile, total omega-6 LCPUFA level was lower in the lowest tertile, and the AA to EPA ratio significantly decreased from the lowest to the highest tertile. The highest tertile of omega-3 LCPUFA had the lowest F2-IsoP to F3-IsoPs ratio, indicating that formation of F3-isoprostanes becomes increasingly dominant over F2-Isoprostane formation at higher omega-3 LCPUFA tissue levels. PCBs and total Hg levels, lipophilic environmental contaminants likely originating from the same marine sources, increased as omega-3 intake augmented.
Omega-3 LCPUFA levels were much higher in older Inuit (age ≥40 yrs; 7.37 ± 3.55%) than in the younger (age <40 yrs; 4.49 ± 2.31%), likely reflecting the higher ingestion of traditional (marine) food items. The plasma F2-IsoP to F3-IsoP ratio was decreased, with both a marginally lower level of F2-IsoPs and a higher level of F3-IsoP. Older individuals were also characterized by higher CRP levels, systolic blood pressure, fasting glucose, cholesterol, and LDL-cholesterol. Mercury and PCBs were higher in older Inuit, as was the level of Se, all likely reflecting the higher consumption of fish and marine food. Using multiple regression analysis, the researchers found that the best predictors of F3-IsoP levels were Hg, omega-3 LCPUFA and EPA intake, as well as smoking and the ratio of AA to EPA levels, the latter two contributing negatively to the variance in F3-IsoP concentrations. The results suggest that several variables, with mutually enforcing and opposing effects, significantly affect F3-isoP levels. Future research taking a mechanistic approach is needed to determine if indeed Hg exposure stimulates F3-isoP formation, whereas smoking depresses this process. In other words, despite a worse cardiometabolic profile in older Inuit, higher Se and F3-isoP levels associated with higher intake of traditional omega-3 LCPUFA-rich foods may be related to some protection from chronic cardiometabolic disease. This was an observational study and no causal links between isoprostane levels or activity with changes in risk factors for cardiometabolic disease can be made. The study, however, provides insight into the qualitative changes in EPA-derived non-enzymatic oxidation products compared to those formed from AA, and suggests that there are fundamental differences between their active formation and their participation in different adaptive tissue reactions. It is currently not clearly understood if and how the body purposefully controls the formation of non-enzymatic oxidation products to regulate different cellular and physiological functions. It is plausible that the body monitors chemical oxidation via the formation of non-enzymatic oxidized fatty acids as an indication of a lack of sufficiency in antioxidants (i.e. vulnerability to oxidation), sensing the need for adaptive corrections in physiological function when oxidative damage to cellular structures becomes appreciable. The results are also compatible with formation of F3-IsoPs being stimulated by mercury, possibly as a protective mechanism. Distinct PUFA-derived isoprostanes and neuroprostanes mediating different hormetic functions in the body cannot be excluded.
Future studies are needed to substantiate some of the underlying assumptions made in this investigation. The evidence that F2-isoprostanes relate to hard cardiovascular disease endpoints, or that the notional potent proinflammatory effects of F2-IsoPs are implicated in cardiovascular pathophysiology, is very limited to date. No evidence for an association between increased F2-IsoP levels and increased fatal or non-fatal coronary heart disease incidence was identified in a prospective cohort study that specifically addressed the relationship between markers of oxidation and cardiovascular disease incidence. Rather, changes in F2-isoprostane levels in tissue fluids may relate better to the risk factors of cardiovascular disease, such as diabetes and smoking. The premise that F3-isoprostanes may have anti-inflammatory and protective effects in human tissues also needs to be evaluated, considering the yet poorly documented causal contributions of F2-isoprostanes to cardiovascular disease. This study also revealed that older Inuit, which more strongly adhere to traditional food sources, display a different pattern of isoprostanes compared to younger generations that have adopted a more Western-type diet, with a much lower omega-3 LCPUFA content. The various parameters that have been identified in this study may help future studies to evaluate the effects of changes in dietary intake of PUFA, particularly in populations that are especially vulnerable to low intake of preformed EPA/DHA and are in the process of abandoning traditional food patterns. Alkazemi D, Jackson RL, Chan HM, Kubow S. Increased F3-isoprostanes in the Canadian Inuit population could be cardioprotective by limiting F2-isoprostane production. J. Clin. Endocrinol. Metab. 2016;101(9):3264-3271. [PubMed] Worth Noting Alkazemi D, Egeland GM, Roberts LJ, 2nd, Kubow S. Isoprostanes and isofurans as non-traditional risk factors for cardiovascular disease among Canadian Inuit. Free Radic. Res. 2012;46(10):1258-1266. [PubMed] Dewailly E, Blanchet C, Lemieux S, Sauve L, Gingras S, Ayotte P, Holub BJ. n-3 Fatty acids and cardiovascular disease risk factors among the Inuit of Nunavik. Am. J. Clin. Nutr. 2001;74(4):464-473. [PubMed] Galano JM, Mas E, Barden A, Mori TA, Signorini C, De Felice C, Barrett A, Opere C, Pinot E, Schwedhelm E, Benndorf R, Roy J, Le Guennec JY, Oger C, Durand T. Isoprostanes and neuroprostanes: total synthesis, biological activity and biomarkers of oxidative stress in humans. Prostaglandins Other Lipid Mediat. 2013;107:95-102. [PubMed] Mori TA. Omega-3 fatty acids and cardiovascular disease: epidemiology and effects on cardiometabolic risk factors. Food Funct. 2014;5(9):2004-2019. [PubMed] Roberts LJ, 2nd, Fessel JP, Davies SS. The biochemistry of the isoprostane, neuroprostane, and isofuran pathways of lipid peroxidation. Brain Pathol. 2005;15(2):143-148. [PubMed] Woodward M, Croft KD, Mori TA, Headlam H, Wang XS, Suarna C, Raftery MJ, MacMahon SW, Stocker R. Association between both lipid and protein oxidation and the risk of fatal or non-fatal coronary heart disease in a human population. Clin. Sci. (Lond) 2009;116(1):53-60. [PubMed]
-
- This study addressed the relationship between omega-3 status in the Inuit and the plasma levels of omega-3 LCPUFA-derived isoprostanes.
- EPA-derived isoprostane levels were positively related to omega-3 fatty acid status, and unrelated to cardiometabolic risk factors, unlike isoprostanes derived from arachidonic acid.
- Older Inuit that adhere more strongly to traditional marine food sources had higher levels of EPA-derived isoprostanes, possibly reflecting a contribution of higher omega-3 intake to reducing cardiometabolic disease risk.
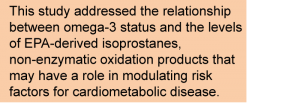
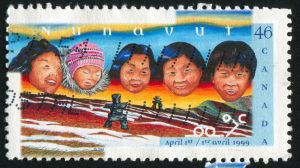
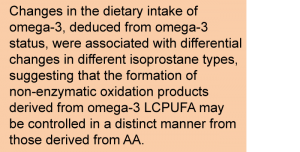
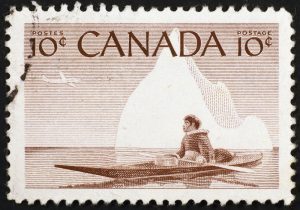
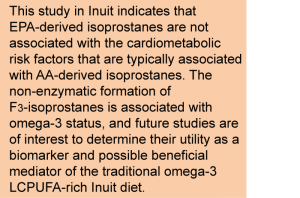