Barbara J Meyer (University of Wollongong, Australia)
Dilys J Freeman (University of Glasgow, UK)
Docosahexaenoic acid (DHA) and polyunsaturated fatty acid (PUFA) metabolism DHA is an omega-3 long chain polyunsaturated fatty acid (n-3 LCPUFA) containing 22 carbons and 6 carbon to carbon double bonds denoted as 22:6n-3. It is an n-3 fatty acid because the first carbon to carbon double bond is on the third carbon from the methyl end of the fatty acid chain: hence the terminology n minus 3 (n-3) because you need to count backwards from the methyl end. We can synthesise most fatty acids ourselves, but there are two essential fatty acids we must obtain from our diet and they are linoleic acid (LA, 18:2n-6) and alpha-linolenic acid (ALA, 18:3n-3). Dietary sources of LA include polyunsaturated margarines/spreads/oils like safflower oil, whereas canola margarine/spreads/oil and walnuts are rich sources of ALA [
1]. Processed foods like snack and takeaway foods contain both LA and ALA [
1]. Human beings can synthesise DHA from ALA via a series of elongation and desaturation steps (see
figure 1). ALA is converted to stearidonic acid (18:4n-3) by the enzyme delta-6 desaturase. The conversion of LA to gamma-linolenic acid (18:3n-6) also uses delta-6 desaturase and given that we consume at least 8 times more LA than ALA in our diets [
2], we have no problems synthesising the n-6 PUFA long chain arachidonic acid (ARA; 20:4n-6) and docosapentaenoic acid (DPA; 22:5n-6) but conversion of ALA right through to DHA is questionable. Women can produce more DHA from ALA than men [
3].
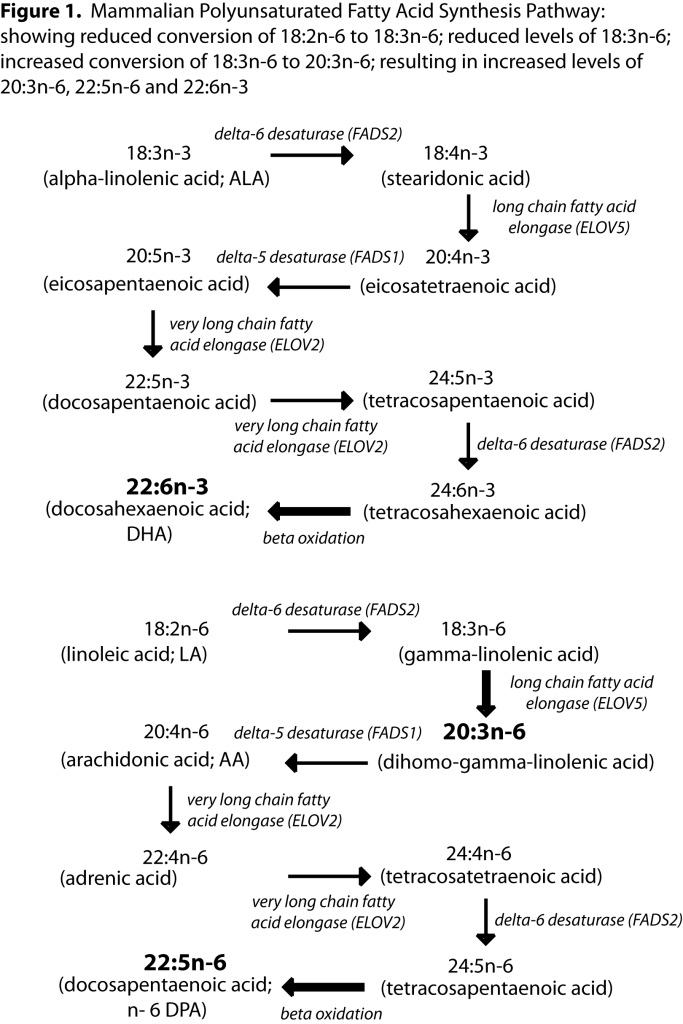
We know that compared to healthy children, DHA levels are 30% lower in children with amino acid metabolism disorders [4] and this is because these children need to severely restrict their dietary protein intake thus limiting/excluding intake of fish/seafood, meat and eggs, all which are foods rich in DHA and ARA. These children do however consume the essential fatty acids LA and ALA from other foods in similar amounts to healthy children. The LA is converted to ARA and incorporated into tissues (e.g. erythrocytes) and the ARA levels do not differ from the healthy children [
4]. This shows that the children with amino acid metabolism disorders have the necessary enzymes to elongate and desaturate LA to ARA through the n-6 pathway. However, these children have DHA levels that are 30% lower than the healthy children meaning they are not able to convert enough ALA to DHA through the n-3 pathway, and therefore DHA should be considered as a semi-essential nutrient (a nutrient that cannot be synthesised in optimal amounts).
DHA composition in the brain DHA accounts for 10% of total fatty acids in the human brain and DHA accounts for 50% of the total PUFA in the brain [
https://www.fatsoflife.com/pufa-april-2016/on-the-contribution-of-plasma-lysophospholipid-and-non-esterified-docosahexaenoic-acid-to-the-brain/]. DHA is a structural component of neuronal cells that enhances membrane fluidity, but it is also involved in neuronal outgrowth and survival [
5]. DHA is mostly bound to phosphatidyl-serine (PS) in the brain [
5] and the levels of DHA in PS is associated with neuronal cell survival [
6]. Both ARA and DHA promote fusion of the membrane phospholipid bilayer thus enabling neurite outgrowth [
7]. The more DHA content of cell membranes, the greater the membrane fluidity [
5]. A good example of scientific evidence from animal studies that supports this membrane fluidity theory is rhodopsin. Rhodopsin is a pigment in the eye that is sensitive to light. Usually when light hits rhodopsin in the eye, elongation of rhodopsin occurs which is necessary for good vision [
8]. This change in conformation requires a fluid membrane. The rhodopsin photocycle is the sequence of structural changes that rhodopsin undergoes in reaction to light and for maximum efficiency requires a high membrane DHA content to keep the membrane fluid and allow the conformational changes to occur. In DHA deficiency, DHA is replaced with n-6 DPA in the eye, conformational changes are not as efficient which affects the rhodopsin photocycle [
9] resulting in reduced visual acuity. Human studies support this in that supplementation with DHA in infants results in increased visual acuity, especially in girl infants [
10].
In DHA deficiency, omega-6 docosapentaenoic acid comes to the rescue In the brain, DHA is also found in PS and has a role to play in maintaining the membrane fluidity required for neuronal function described above. In situations where DHA deficiency occurs and DHA supply in the brain is limited, n-6 DPA can substitute for DHA in PS in the brain in a similar manner to what occurs in rhodopsin in the eye, as explained above [
11]. This means membrane fluidity is maintained at an acceptable, but by no means optimal, level but it allows brain function to be maintained in the face of DHA deficiency [
6]. n-6 DPA is not quite as good as DHA at making fluid PS membranes but it is the second-best option and means PS membranes continue to be formed in the brain [
12]. When DHA is replete, DHA displaces n-6 DPA from neuronal cells and function returns to normal.
Requirement for DHA in very early pregnancy; particularly at the critical time of the neural tube closure The critical requirement for DHA for fetal brain development, and the low efficiency of conversion of ALA to DHA, is a metabolic problem that needs to be overcome during pregnancy. There is rapid accumulation of DHA and ARA during the third trimester of pregnancy [
13]. We have recently shown that DHA concentrations increase in very early pregnancy [
14]. Our very early pregnancy study [
14] involved women undergoing assisted conception to achieve accurately timed peri-conceptual sampling. Women with a regular natural menstrual cycle to avoid the effects of exogenous hormones underwent frozen embryo transfer. We took blood samples prior to embryo transfer and at days 18, 29 and 45 after transfer. A positive fetal heartbeat at day 45 was our measure of successful pregnancy. Maternal DHA pre-pregnancy levels were 145nmol/ml plasma (1.9% DHA of total plasma fatty acids). Our data shows that the mother increases her plasma DHA concentration by day 29 and it continues to rise to day 45 (190nmol/ml plasma; 2.5% DHA of total plasma fatty acids). DHA was the only maternal plasma fatty acid that had a positive rate of change across the first 45 days; 0.12nmol DHA/ml plasma per day at day 18 increasing to 1.6nmol DHA/ml plasma per day at day 29 and 1.8nmol DHA/ml plasma per day at day 45. Notably the greatest rate of change was between days 18 and 29, when the neural tube closes. When looking at the amount of fatty acid released into the plasma over 45 days (as assessed by the incremental area under the curve), DHA and n-6 DPA were the only fatty acids with positive values of 632 and 42 nmol/ml plasma in 45 days respectively [
14]. We were unable to measure delta-6 desaturase activity directly as we could not collect maternal liver samples, so we used a surrogate of delta-6 desaturase activity; namely the ratio of 20:3n-6 to 18:2n-6. At day 29, the delta-6 desaturase activity correlated positively (R
2 adj = 0.41, p=0.0002) with the DHA rate of change (nmol/ml per day) [
14]. There were no such significant correlations at day 18 or day 45, which suggests that the synthesis of DHA was most important between day 18 and 29, the time of neural tube closure. There was a significant 10% reduction in plasma LA from pre-pregnancy to day 18 in the pregnant women but there was no 10% reduction in LA in the non-pregnant women [
14]. There was also a 28% reduction in gamma-linolenic acid (18:3n-6) and a 28% increase in dihomo-gamma-linolenic acid (20:3n-6) and a 42% increase in n-6 DPA. The 10% reduction in LA and the accelerated increase in DHA between days 18 and 29 of the study being correlated with delta-6 desaturase suggests an increased ability to convert ALA to DHA (i.e. the n-3 elongation and desaturation pathway going ahead). Similar findings were seen in the third trimester of pregnancy, where low LA levels have been associated with higher DHA levels in mothers in their third trimester of pregnancy as well as their newborns, and the low LA placental transport allowed for more ALA to be converted to DHA in the fetus [
15].
Twins need triple the amount of DHA and n-6 DPA The amount of DHA, as assessed by the incremental area under the curve (iAUC), in twin pregnancies was at least 3-fold higher than singleton pregnancies (1341 versus 384 nmol/ml over 45 days) (
figure 2). Similarly, n-6 DPA was 4-fold higher in twin pregnancies compared with singleton pregnancies (96 versus 23nmol/ml over 45 days [
14].
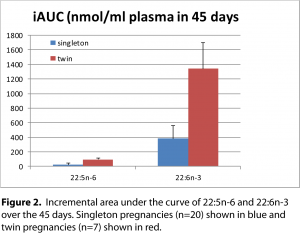
In twin pregnancies, there is a greater maternal supply of DHA and n-6 DPA, fatty acids that are required for neurodevelopment. These results would suggest that n-6 DPA is increased in case there is not enough DHA for neuronal development, similar to the animal studies and rhodopsin as described earlier. We interpret these results as n-6 DPA is being increased as a back-up in case there is not enough DHA available at this critical time of neurodevelopment.
Low maternal plasma LA levels at day 18 predict successful pregnancy at day 45 From all the metabolic data that we collected in this study there were only three maternal variables at day 18 that predicted successful pregnancy at day 45; namely reduced plasma LA, increased insulin and increased erythrocyte saturated fatty acids [
14]. The reduction in LA would allow greater conversion of ALA to DHA. The higher insulin would release fatty acids from maternal fat stores and the increased erythrocyte saturated fatty acid levels is probably a marker of this and collectively it would suggest that necessary fuel is being supplied to growing embryo. In conclusion (refer to timeline below), in very early pregnancy the mother appears to adapt metabolically in order to provide enough DHA and n-6 DPA at the critical time when the neural tube closes.
References: 1 Ollis TE, Meyer BJ, Howe PR. Australian food sources and intakes of omega-6 and omega-3 polyunsaturated fatty acids.
Annals of Nutrition & Metabolism 1999;43(6):346-355. (
link) 2 Meyer BJ. Australians are not meeting the recommended intakes for omega-3 long chain polyunsaturated fatty acids: Results of an analysis from the 2011-2012 National Nutrition and Physical Activity Survey.
Nutrients 2016;8(3):111. (
link) 3 Burdge GC, Wootton SA. Conversion of alpha-linolenic acid to eicosapentaenoic, docosapentaenoic and docosahexaenoic acids in young women.
The British Journal of Nutrition 2002;88(4):411-420. (
link) 4 Vlaardingerbroek H, Hornstra G, de Koning TJ, Smeitink JA, Bakker HD, de Klerk HB, Rubio-Gozalbo ME. Essential polyunsaturated fatty acids in plasma and erythrocytes of children with inborn errors of amino acid metabolism.
Molecular Genetics and Metabolism 2006;88(2):159-165. (
link) 5 Parletta N, Milte CM, Meyer BJ. Nutritional modulation of cognitive function and mental health.
The Journal of Nutritional Biochemistry 2013;24(5):725-743. (
link) 6 Kim HY. Novel metabolism of docosahexaenoic acid in neural cells.
The Journal of Biological Chemistry 2007;282(26):18661-18665. (
link) 7 Darios F, Davletov B. Omega-3 and omega-6 fatty acids stimulate cell membrane expansion by acting on syntaxin 3.
Nature 2006;440(7085):813-817. (
link) 8 Alves ID, Salgado GFJ, Salamon Z, Brown MF, Tollin G, Hruby VJ. Phosphatidylethanolamine enhances rhodopsin photoactivation and transducin binding in a solid supported lipid bilayer as determined using plasmon-waveguide resonance spectroscopy.
Biophysical Journal 2005;88(1):198-210. (
link) 9 Niu SL, Mitchell DC, Litman BJ. Optimization of receptor-G protein coupling by bilayer lipid composition II: formation of metarhodopsin II-transducin complex.
The Journal of Biological Chemistry 2001;276(46):42807-42811. (
link) 10 Innis SM, Friesen RW. Essential n-3 fatty acids in pregnant women and early visual acuity maturation in term infants.
The American Journal of Clinical Nutrition 2008;87(3):548-557. (
link) 11 Kim H-Y, Bigelow J, Kevala JH. Substrate preference in phosphatidylserine biosynthesis for docosahexaenoic acid containing species.
Biochemistry 2004;43(4):1030-1036. (
link) 12 Garcia MC, Ward G, Ma Y-C, Salem N, Kim H-Y. Effect of docosahexaenoic acid on the synthesis of phosphatidylserine in rat brain microsomes and C6 glioma cells.
Journal of Neurochemistry 1998;70(1):24-30. (
link) 13 Makrides M, Gibson RA. Long-chain polyunsaturated fatty acid requirements during pregnancy and lactation.
The American Journal of Clinical Nutrition 2000;71(1 Suppl):307S-311S. (
link) 14 Meyer BJ, Onyiaodike CC, Brown EA, Jordan F, Murray H, Nibbs RJ, Sattar N, Lyall H, Nelson SM, Freeman DJ. Maternal plasma DHA levels increase prior to 29 days post-lh surge in women undergoing frozen embryo transfer: a prospective, observational study of human pregnancy.
The Journal of Clinical Endocrinology and Metabolism 2016;101(4):1745-1753. (
link) 15 Novak EM, King DJ, Innis SM. Low linoleic acid may facilitate Delta6 desaturase activity and docosahexaenoic acid accretion in human fetal development.
Prostaglandins, Leukotrienes, and Essential Fatty Acids 2012;86(3):93-98. (
link)