On the Contribution of Plasma Lysophospholipid and Non-Esterified Docosahexaenoic Acid to the Brain
Richard P. Bazinet Associate Professor, Canada Research Chair in Brain Lipid Metabolism Department of Nutritional Sciences, Faculty of Medicine, University of Toronto FitzGerald Building, 150 College Street, Room 306 Toronto, ON, Canada M5S 3E2 Email - richard.bazinet@utoronto.ca The brain has a lot of docosahexaenoic acid
The brain is particularly enriched with the polyunsaturated fatty acid (PUFA) docosahexaenoic acid (DHA; 22:6n-3) while being almost devoid of other omega-3 PUFA, such as eicosapentaenoic acid (EPA). Within the brain DHA makes up around 10% of the total fatty acids and about 50% of the PUFA, corresponding to a concentration of about 10,000 nmol/g or about 5 grams in the whole adult brain1. DHA is especially enriched in the grey matter and the synapses where it participates in signal transduction, either directly or upon bioconversion to a series of lipid mediators including protectin D1 and docosahexaenoyl ethanolamide. Collectively, DHA and its metabolites have been shown to regulate cell survival, cell growth and neuroinflammation within the brain2-4. Because the brain cannot synthesize DHA de novo, the brain relies on the uptake of DHA from the blood to replace the DHA consumed in metabolic reactions. Lipoproteins The plasma pools and mechanisms by which fatty acids such as DHA enter the brain have long been debated5-7. Plasma pools thought to supply the brain with DHA included lipoproteins, especially low-density lipoproteins (LDL), lyso-phospholipids containing DHA and non-esterified DHA. In order to test the hypothesis that the LDL receptor was necessary for maintaining brain DHA levels, we measured DHA concentrations in wild type and LDL receptor knock-out mice8. However, a bit to our surprise, there was no difference in brain DHA concentrations between knock-out and wild type controls. This does not mean that the LDL receptor is not involved in maintaining brain DHA levels or in the transport of DHA, but rather that it is not necessary and that other mechanisms are sufficient. Interestingly, VLDL receptor knock-out mice also did not have lower brain DHA levels than wild type controls9. While using knock-outs can provide useful information, even if we lowered DHA in the absence of a candidate transporter, it would not have proved that the candidate was directly important for transport or uptake, nor would the quantitative relevance have been known. Non-esterified docosahexaenoic acid Stanley Rapoport’s laboratory developed a method to quantitate the rate of uptake of plasma non-esterified fatty acids into the brain10. The method involves infusing a labelled fatty acid tracer intravenously into the unanaesthetized rodent for 5 minutes upon which the label is measured in the brain. The short duration is important for several reasons, including that if the infusion continues for too long, non-esterified fatty acids, including DHA, are taken up by the liver and secreted into the lipoprotein pool esterified to phospholipids and other glycerides as well as in cholesteryl esters. Thus, upon detecting the tracer fatty acid in the brain, it would be unclear which plasma pool(s) were contributors to brain uptake; however, this problem is avoided with the acute infusion model. During the infusion blood is taken and the amount of the tracer in the brain is corrected for the area under the curve of the infused tracer giving a unidirectional incorporation coefficient. By multiplying the incorporation coefficient by the plasma concentration of the non-esterified fatty acid, the uptake rate into the brain or brain phospholipids can be determined. This method has been used by several labs to determine the rate of uptake of DHA, arachidonic acid, EPA, erucic acid, linolenic acid, alpha-linolenic acid, docosapentaenoic acid and palmitic acid into the brain11-16. While this approach is quantitative, it was necessary to figure out if the amount entering the brain from the plasma non-esterified DHA pool was a major or minor contributor to the brain. One piece of evidence that the non-esterified DHA pool was a major contributor to brain DHA was that the rate of DHA entry into the brain in control animals overlapped with the rate of brain consumption of DHA in animals that were either on a diet deprived of or containing n-3 PUFA17. Because the rate of DHA entering the brain was approximating the rate of DHA consumption, and brain DHA levels are constant in adult rodents, this provided evidence that the non-esterified pool is a major contributor to brain DHA uptake18. Furthermore, consistent with this observation, while the brain can elongate some alpha-linolenic acid to DHA, the rate of the reaction was quantified in vivo and found to be just a very small fraction of the DHA uptake rate from the non-esterified pool14, a finding that was later also demonstrated with the conversion of EPA to DHA15. Lyso-phosphatidylcholine Lyso-phosphatidylcholine (LPC) containing DHA can be present in the “free” plasma pool or as part of lipoproteins. Earlier work in squirrel monkeys had suggested that plasma LPC esterified to palmitic acid was an important source of both phosphatidylcholine fatty acid and choline for the brain19. This work was followed up and significantly advanced by Michel Lagarde’s group who demonstrated that several LPCs (palmitic, oleic, linoleic and arachidonic acids) not only entered the brain, but upon an acute intravenous perfusion of labeled LPC, more labelled fatty acid was present in the brain as compared to administration of the non-esterified tracer20. Interestingly, their work also appeared to demonstrate that arachidonic acid esterified to LPC had a longer plasma half-life than non-esterified arachidonic acid. This work was followed up by demonstrating that upon an intravenous perfusion, more labelled DHA was present in the brain when the tracer was given as LPC-DHA as compared to non-esterified DHA21. Furthermore, this paper demonstrated (although not tested statistically) that several LPCs, including LPC-DHA, had longer plasma half-lives
than non-esterified DHA. While this earlier work might have seemed to be at odds with the observation that non-esterified DHA appeared to be entering the brain at the same rate by which DHA was being consumed in the brain, there were several important differences in the studies. Firstly, the work on LPC-DHA was mostly performed in young (20-day old) rats, which might be important given that the young brain has the additional burden of accreting DHA. Thus, it is possible that additional mechanisms of uptake are present in the young rat as compared to the adult. Also, the terms “preferential” or “more efficiency” were used to describe the uptake results20-22, which was reasonable given that more labelled DHA was present in the brain upon intravenous LPC-DHA administration compared to non-esterified DHA. The next papers Building, in part, on the work of Lagarde and colleagues, David Silver’s laboratory performed a series of elegant experiments in wildtype and Mfsd2a knock-out mice23. Mfsd2a knock-out mice had about 8 percent DHA in brain phospholipids, while wild type mice had close to 20 percent. This and a series of other observations led them to explore the relationship between Mfsd2a and LPCs, including LPC-DHA as well as non-esterified DHA. While indirect genomic and biochemical evidence was considered supportive of an important role for LPC-DHA and the brain, the only direct evidence related to uptake presented were studies examining the appearance of labelled DHA upon non-esterified DHA and LPC-DHA injection. In these experiments, which were broadly similar to earlier studies, tracers of LPC-DHA and non-esterified DHA were perfused intravenously and the appearance of the label in the brain was measured two hours later. Again, similar to the previous work, the amount of labelled DHA in the brain after two hours was higher upon intravenous LPC-DHA administration than non-esterified DHA administration. However, surprisingly (at least to us) this paper concluded that LPC-DHA was the major source of DHA supplying the brain. It was not clear if the tracer experiments and/or the indirect genomic and biochemical data were the foundation of this conclusion. Nevertheless, and ignoring that within about 15 minutes, let alone two hours after intravenous administration of the tracers, they would be present in multiple plasma pools and it would be impossible to determine which pool had contributed DHA to the brain, there were no experiments comparing the rate of entry of the two candidate pools of DHA into the brain. This seemed especially important given the longer plasma half-life of LPC-DHA (several minutes) compared to unesterified DHA (about 30 seconds). If a tracer has a longer half-life, its entry into the brain could be slower, but given the length of the experiment it would have more opportunity to enter the brain. For instance, in about 2.5 minutes, the non-esterified DHA tracer would be virtually cleared from the plasma while there would still be about 50% of the dose of the LPC-tracer circulating and able to enter brain. Furthermore, one has to remember that while the tracer levels are not at steady-state, the unlabeled (tracee) pools would be at steady-state and not approaching zero.
In order to shed light on this controversy we conducted a series of kinetic experiments24. First, we replicated the finding that plasma non-esterified DHA entered the brain at a rate approximating DHA being consumed in the brain. Second, we gave labelled DHA orally and observed its appearance in many plasma pools, including the LPC-DHA pool and the brain. In this experiment we were able to demonstrate that applying the experimentally derived uptake coefficient for non-esterified DHA to the oral experiment we could explain most, if not all, of the label in the brain. Collectively, the results of these experiments left little room for the uptake of other plasma DHA pools into the brain. We then attempted to replicate the study from the Silver’s group. Two hours upon intravenous administration of labelled LPC or non-esterified DHA, there was more labelled DHA in the brain of animals that received LPC-DHA. However, we also measured other plasma DHA pools during the study. As we expected, labelled DHA, under both conditions, appeared in numerous plasma pools during the two hours, making it impossible to tell which pool(s) had contributed DHA to the brain. Furthermore, we calculated that the amount of labelled LPC-DHA in the plasma that the brain is exposed to was about five times more than non-esterified DHA during the course of the experiment, entirely consistent with the longer plasma half-life of LPC-DHA. When we for the exposure to the brain modelled the net rate of uptake, it was evident that the rate of non-esterified DHA into the brain was many times higher than LPC-DHA. In our last experiment, in part to avoid the issues with the longer experiments, we continuously infused labelled LPC-DHA or non-esterified DHA for five minutes and applied the modelling developed by Stanley Rapoport. Again, consistent with the longer half-life of LPC-DHA than non-esterified DHA, its contribution to plasma radioactivity was higher and it continued to rise during the study (because it is not being cleared as fast). Finally, we found that the plasma non-esterified pool was supplying about 10 times more DHA to the brain than LPC-DHA. But LPC-DHA is still very important. Our findings that non-esterified DHA is the major plasma pool supplying the brain do not negate the importance of the LPC-DHA pool. Firstly, as has been known for over 50 years LPCs are important sources of choline to the brain. Secondly, even though LPC-DHA is not the major source of brain DHA under normal physiological conditions, it is still a viable therapeutic approach to target the brain with DHA. While it may not seem intuitive, a higher percentage of exogenously administered (i.e. a bolus intravenous dose) LPC-DHA will reach the brain as compared to non-esterified DHA over time. This is because the administered LPC-DHA will circulate in the plasma and continue to be taken up by the brain long after the administered non-esterified DHA has been taken up (note: in vivo the plasma levels are maintaining at a steady-state). Thus, the use of LPC-DHA is a reasonable approach for targeting the brain. In fact, much success has been ascribed to LPC-DHA, relative to non-esterified DHA, in preclinical models of stroke25. Studies are currently making more stable derivatives of LPC-DHA which may enhance its suitability for delivery to the brain26,27 and an obvious area of research would be to try and target the plasma LPC-DHA pool via oral delivery. It will also be important to examine these mechanisms during times of increased brain DHA demand (i.e. brain growth) to test the relative contribution of plasma pools. There may very well be multiple mechanisms that are upregulated to supply the brain with DHA during early brain development. However, because the uptake of non-esterified DHA has been estimated in adult humans using positron emission tomography to be about 2.4-3.8 mg per day28,29, we can now look at the partitioning of DHA between the brain and tissues as well as the role of diet, disease and other physiological conditions in maintaining this contribution to the brain30. References.
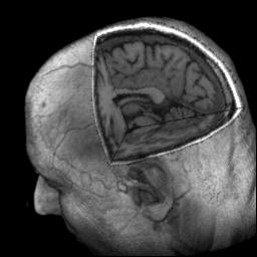
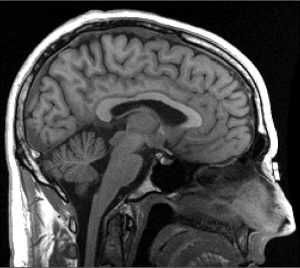
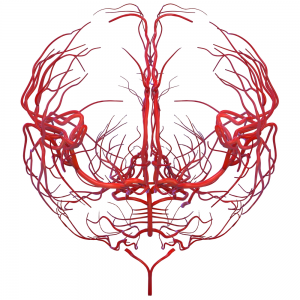
- Bazinet RP, Laye S. Polyunsaturated fatty acids and their metabolites in brain function and disease. Nat Rev Neurosci 2014;15:771-85.
- Bazan NG, Molina MF, Gordon WC. Docosahexaenoic acid signalolipidomics in nutrition: significance in aging, neuroinflammation, macular degeneration, Alzheimer's, and other neurodegenerative diseases. Annu Rev Nutr 2011;31:321-51.
- Salem N, Jr., Litman B, Kim HY, Gawrisch K. Mechanisms of action of docosahexaenoic acid in the nervous system. Lipids 2001;36:945-59.
- Rashid MA, Katakura M, Kharebava G, Kevala K, Kim HY. N-Docosahexaenoylethanolamine is a potent neurogenic factor for neural stem cell differentiation. J Neurochem 2013;125:869-84.
- Hamilton JA, Kamp F. How are free fatty acids transported in membranes? Is it by proteins or by free diffusion through the lipids? Diabetes 1999;48:2255-69.
- Rapoport SI, Chang MC, Spector AA. Delivery and turnover of plasma-derived essential PUFAs in mammalian brain. J Lipid Res 2001;42:678-85.
- Mitchell RW, Hatch GM. Fatty acid transport into the brain: of fatty acid fables and lipid tails. Prostaglandins Leukot Essent Fatty Acids 2011;85:293-302.
- Chen CT, Ma DW, Kim JH, Mount HT, Bazinet RP. The low density lipoprotein receptor is not necessary for maintaining mouse brain polyunsaturated fatty acid concentrations. J Lipid Res 2008;49:147-52.
- Rahman T, Taha AY, Song BJ, et al. The very low density lipoprotein receptor is not necessary for maintaining brain polyunsaturated fatty acid concentrations. Prostaglandins Leukot Essent Fatty Acids 2010;82:141-5.
- Robinson PJ, Noronha J, DeGeorge JJ, Freed LM, Nariai T, Rapoport SI. A quantitative method for measuring regional in vivo fatty-acid incorporation into and turnover within brain phospholipids: review and critical analysis. Brain Res Brain Res Rev 1992;17:187-214.
- Golovko MY, Murphy EJ. Uptake and metabolism of plasma-derived erucic acid by rat brain. J Lipid Res 2006;47:1289-97.
- Igarashi M, Kim HW, Gao F, Chang L, Ma K, Rapoport SI. Fifteen weeks of dietary n-3 polyunsaturated fatty acid deprivation increase turnover of n-6 docosapentaenoic acid in rat-brain phospholipids. Biochim Biophys Acta 2012;1821:1235-43.
- DeMar JC, Jr., Lee HJ, Ma K, et al. Brain elongation of linoleic acid is a negligible source of the arachidonate in brain phospholipids of adult rats. Biochim Biophys Acta 2006;1761:1050-9.
- Demar JC, Jr., Ma K, Chang L, Bell JM, Rapoport SI. alpha-Linolenic acid does not contribute appreciably to docosahexaenoic acid within brain phospholipids of adult rats fed a diet enriched in docosahexaenoic acid. J Neurochem 2005;94:1063-76.
- Chen CT, Domenichiello AF, Trepanier MO, Liu Z, Masoodi M, Bazinet RP. The low levels of eicosapentaenoic acid in rat brain phospholipids are maintained via multiple redundant mechanisms. J Lipid Res 2013;54:2410-22.
- Chen CT, Green JT, Orr SK, Bazinet RP. Regulation of brain polyunsaturated fatty acid uptake and turnover. Prostaglandins Leukot Essent Fatty Acids 2008;79:85-91.
- DeMar JC, Jr., Ma K, Bell JM, Rapoport SI. Half-lives of docosahexaenoic acid in rat brain phospholipids are prolonged by 15 weeks of nutritional deprivation of n-3 polyunsaturated fatty acids. J Neurochem 2004;91:1125-37.
- Rapoport SI, Rao JS, Igarashi M. Brain metabolism of nutritionally essential polyunsaturated fatty acids depends on both the diet and the liver. Prostaglandins Leukot Essent Fatty Acids 2007;77:251-61.
- Illingworth DR, Portman OW. The uptake and metabolism of plasma lysophosphatidylcholine in vivo by the brain of squirrel monkeys. Biochem J 1972;130:557-67.
- Thies F, Delachambre MC, Bentejac M, Lagarde M, Lecerf J. Unsaturated fatty acids esterified in 2-acyl-l-lysophosphatidylcholine bound to albumin are more efficiently taken up by the young rat brain than the unesterified form. J Neurochem 1992;59:1110-6.
- Thies F, Pillon C, Moliere P, Lagarde M, Lecerf J. Preferential incorporation of sn-2 lysoPC DHA over unesterified DHA in the young rat brain. Am J Physiol 1994;267:R1273-9.
- Lagarde M, Bernoud N, Brossard N, et al. Lysophosphatidylcholine as a preferred carrier form of docosahexaenoic acid to the brain. J Mol Neurosci 2001;16:201-4; discussion 15-21.
- Nguyen LN, Ma D, Shui G, et al. Mfsd2a is a transporter for the essential omega-3 fatty acid docosahexaenoic acid. Nature 2014;509:503-6.
- Chen CT, Kitson AP, Hopperton KE, et al. Plasma non-esterified docosahexaenoic acid is the major pool supplying the brain. Sci Rep 2015;5:15791.
- Chauveau F, Cho TH, Perez M, et al. Brain-targeting form of docosahexaenoic acid for experimental stroke treatment: MRI evaluation and anti-oxidant impact. Curr Neurovasc Res 2011;8:95-102.
- Lagarde M, Hachem M, Bernoud-Hubac N, Picq M, Vericel E, Guichardant M. Biological properties of a DHA-containing structured phospholipid (AceDoPC) to target the brain. Prostaglandins Leukot Essent Fatty Acids 2014.
- Hachem M, Geloen A, Van AL, et al. Efficient Docosahexaenoic Acid Uptake by the Brain from a Structured Phospholipid. Mol Neurobiol 2015.
- Umhau JC, Zhou W, Carson RE, et al. Imaging incorporation of circulating docosahexaenoic acid into the human brain using positron emission tomography. J Lipid Res 2009;50:1259-68.
- Umhau JC, Zhou W, Thada S, et al. Brain Docosahexaenoic Acid [DHA] Incorporation and Blood Flow Are Increased in Chronic Alcoholics: A Positron Emission Tomography Study Corrected for Cerebral Atrophy. PLoS One 2013;8:e75333.
- Domenichiello AF, Kitson AP, Bazinet RP. Is docosahexaenoic acid synthesis from alpha-linolenic acid sufficient to supply the adult brain? Prog Lipid Res 2015;59:54-66.